Metabolic Modeling and Genetic Engineering of Enhanced Anaerobic Microbial Ethylene Synthesis
Authors:
Sarah, J. Young1, Adrienne B. Narrowe3, Kamal M. Deep1, Elizabeth Morgan1, Rebecca Daly3, Mikayla S. Borton3, Ethan King2, Kelly C. Wrighton3, William R. Cannon2, and Justin A. North1* ([email protected])
Institutions:
1The Ohio State University; 2Pacific Northwest National Laboratory; and 3Colorado State University
Goals
To develop robust and optimized anaerobic ethylene pathways in photosynthetic and lignocellulosic bacteria for high-yield conversion of renewable CO2 and lignocellulose into bioethylene. This will be accomplished by:
1: First, bioinformatically mining and experimentally screening methylthio-alkane reductase homologs, SAM hydrolase homologs, and alcohol dehydrogenase homologs from cultivated and uncultivated organisms for functional enzymes that enhance ethylene yields.
2: Next, constructing and employing predictive systems-level models of ethylene production. Using a physics-based R. rubrum model, researchers predict enzymes that participate in competing or supporting pathways and are thus targets for selection studies to increase ethylene yields.
3: Finally, researchers metabolically engineer bacteria for enhanced, sustained ethylene production from CO2 and lignocellulose. Researchers assemble the best-performing genes under control of optimized active transcription elements on a modular DNA fragment in a combinatorial manner with guidance from predictive models (Aim 2).
Abstract
Previously researchers have detailed a pathway in phototrophic bacterium, Rhodospirillum rubrum that produces ethylene in the absence of oxygen from methionine and ATP (Fig. 1; North et al. 2020). Traditional ethylene production involves energy intensive cracking of petroleum fossil fuels to meet the 300 million metric ton annual demand. Thus, a sustainable microbial platform for the renewable production of ethylene is urgently needed. The goal of this project is to optimize this anaerobic ethylene production pathway, as outlined above.
Enzyme Screens: Experimentally verified amino acid sequences for each reaction (Fig. 1, *) were queried against Uniref, KEGG, GenBank, and JGI-IMG databases using an e-value cutoff of 1e-10. Sequences were also searched using BLAST (e-value cutoff of 1e-10) in a curated set of assembled genome databases spanning soils, rivers, and the human gut. Combined sequences from all databases were dereplicated using CD-HIT to remove identical sequences. Methylthio-alkane reductase genes were synthesized by the JGI DNA synthesis program, and SAM hydrolases and alcohol dehydrogenases synthesis in process. Insertion of the methylthio-alkane reductase genes into an R. rubrum native methylthio-reductase deletion strain revealed sequences from closely related alphaprotebacteria like Rhodoblastus sphagnicola increase ethylene synthesis from 2-methylthioethanol up to 2-fold. Furthermore, these screens revealed sequences from clostridia, bacilli, negativicutes, and fibrobacter species that are also methylthio-alkane reductases, expanding the understanding of the diversity of organisms that can synthesis hydrocarbons from volatile organic sulfur compounds. In parallel to these enzyme screens, researchers have further enumerated the number of substrates and reactions catalyzed, revealing that propane, propylene, and butane can also be synthesized.
Physics-based Modeling: In order to ensure that the model of cell metabolism is complete enough to capture the important dynamics for ethylene production, researchers iteratively built and tested the model on four photoheterotrophic growth conditions: growth on fumarate, malate, acetate and ethanol. Testing and refining the model on a variety of growth conditions helps to ensure that the principles discovered regarding the metabolic processes are general and not artifacts of an incomplete model. Researchers have now tested the model with each growth condition and a range of redox states and compared the results to various experimental studies. Summarizing the results, the model predicts that flux through phosphoenolpyruvate carboxy kinase (PEP-CK) during growth on malate and fumarate is thermodynamically feasible to synthesize phosphoenol-pyruvate in lower glycolysis, as observed experimentally (McCully and McKinlay 2016). However, the model indicates that the reductive TCA (rTCA) cycle is the thermodynamically favored mechanism for pyruvate synthesis to support gluconeogenesis, amino acid, and de novo nucleotide synthesis. In contrast, during growth on acetate and ethanol, the thermodynamically preferred route to synthesize pyruvate utilizes the ethylmalonyl-CoA pathway for acetyl-CoA synthesis followed by reductive carboxylation to pyruvate by pyruvate ferredoxin oxidoreductase. To predict ethylene production, the computational model has been analyzed with metabolic control analysis (concentration control coefficients) and with computational protein knock-down (reduced pathway activity) and overexpression (increased pathway activity) to predict which enzymes need to be up or down regulated to enhance ethylene production. Initial results have had very good agreement with parallel experimental work.
Metabolic Engineering: In Rhodospirillum rubrum at least 6 ethylene pathway enzymes are under transcriptional regulation of SalR, a member of LysR family transcription factors. SalR and thus ethylene synthesis activity is sensitive to available exogenous sulfur, such that ethylene synthesis is turned off at sulfur concentrations ≥ 200 μM. Instead of replacing the promoter for each gene, researchers are taking a more efficient approach by engineering SalR expression and activity to activate ethylene synthesis. When SalR is constitutively expressed the engineered strain continuously produces ethylene, even in presence of ≥ 500 uM of exogeneous sulfate and methionine. However, exogenous cysteine still inhibits ethylene similar to when SalR is expressed from its native promoter suggesting cysteine is a SalR effector molecule. Through predictive structural modeling (Alpha Fold) researchers have identified residues for substitution studies.
Image
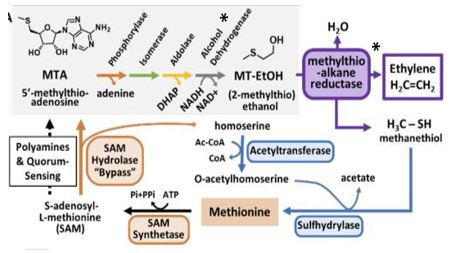
Fig. 1 Anaerobic Ethylene Cycle for microbial synthesis of ethylene. Optimal enzymes for reactions indicated by (*) are identified by gene synthesis and screening. The ethylene pathway plus central carbon metabolism and DNA/RNA/protein synthesis is being assessed by physics-based modeling to identify metabolic bottlenecks of burdens.
References
North, J. A., et al. 2020. “A Nitrogenase-like Enzyme System Catalyzes Methionine, Ethylene, and Methane Biogenesis.” Science 369(6507), 1094–8. DOI: 10.1126/science.abb6310.
McCully A. L., and J. B. McKinlay 2016. “Disrupting Calvin Cycle Phosphoribulokinase Activity in Rhodopseudomonas palustris Increases the H2 Yield and Specific Production Rate Proportionately.” International Journal of Hydrogen Energy 41(7), 4143–9.
Funding Information
This project is supported by the U.S. Department of Energy’s Office of Biological and Environmental Research under contracts DE-SC0022091 and DE-SC0019338 (Ohio State University) and 78266 (PNNL).